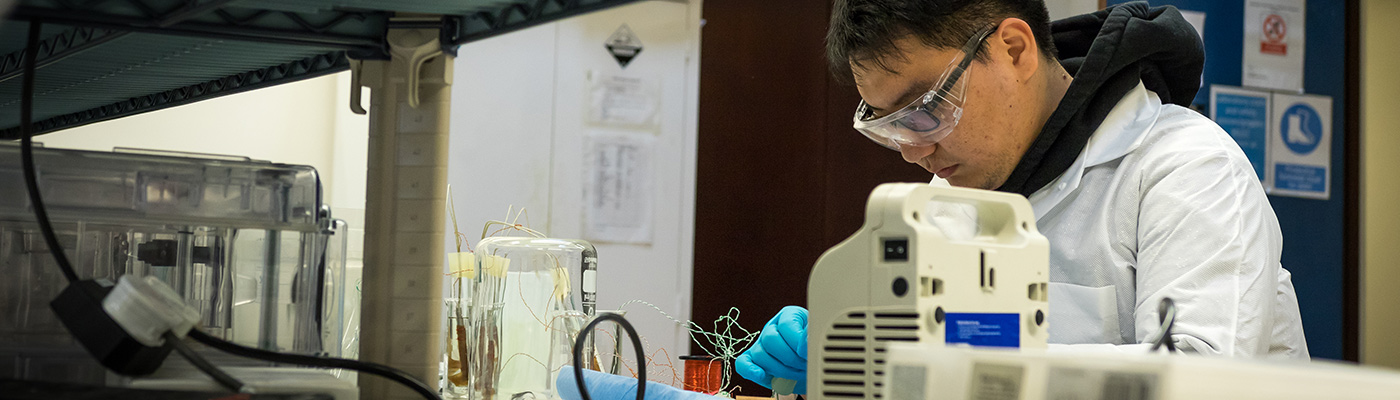
Why study here?
The Department of Electrical and Electronic Engineering at Manchester is one of the largest in the UK, with a reputation for teaching and research excellence.
Why Electrical and Electronic Engineering at Manchester?
- Ranked 4th in the UK for electrical and electronic engineering (QS World University Rankings, 2023)
- Experience our brand new £400m Home of Engineering and Materials Science with state-of-the-art facilities and study spaces.
- The largest high-voltage lab in the UK.
We've been educating electrical and electronic engineers for more than a century.
Today the Department boasts outstanding facilities - such as those at the National Instruments Undergraduate Teaching Lab - and excellent links with industry, including world-renowned companies such as Siemens, Rolls-Royce and Google.
What's more, all of our courses are accredited by the Institution of Engineering and Technology.
As a student you'll join a close, welcoming community with a real emphasis on fun. You can join a host of clubs and societies, including:
- The E4C Electronics Club
- The Electrical and Electronic Engineering Society
- The Robotics Society.
Our postgraduate students benefit from access to wonderful facilities and a huge breadth of research expertise, and - last but not least - our second-year undergraduate students can compete in our famous robot race day:
Discover Manchester
Explore the countless benefits of the Manchester student experience.
Our facilities
Explore the outstanding facilities you'll have access to as a student or researcher in our Department.
Career opportunities
Open up any number of exciting career paths with a degree from the Department.